Asking the Soft Questions
Time to change your idea of what a robot looks like
By Kirsten Janson
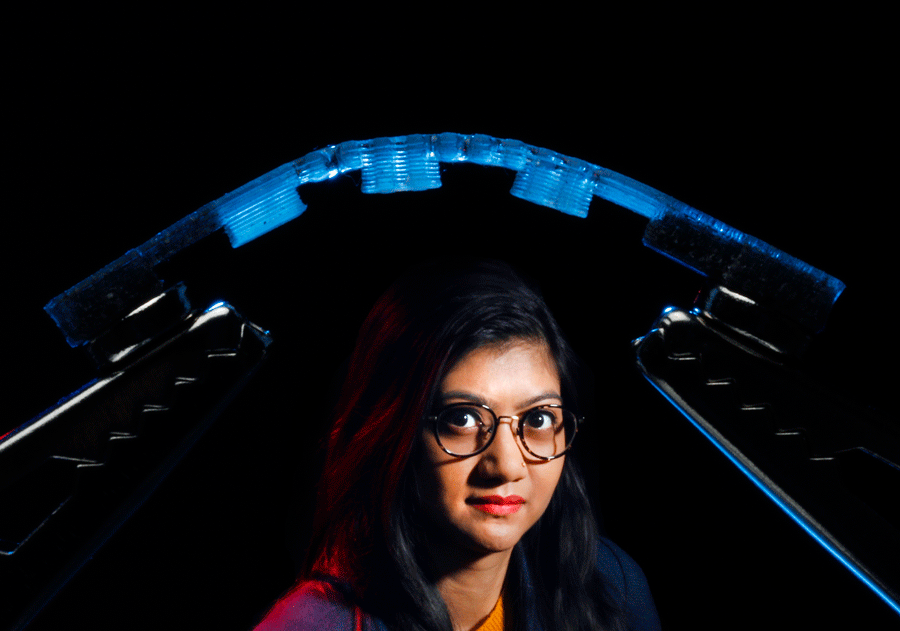
Erina Joyee has a collection of inchworms. Not the fluorescent green ones that crawl on trees. Hers are clear and crawl on chicken skin.
They’re robots.
In recent months, Joyee, a PhD candidate in industrial engineering, has been busy testing the maneuverability of her newest inchworm, a fully 3D-printed robot prototype that scoots around on a magnetic field and mimics the real live insect’s push-pull crawling mechanism. The robot lives in UIC’s Additive Manufacturing Laboratory for now, but someday it could be used to deliver drugs or perform in-vivo imaging inside the human body.
Robots like Joyee’s are part of a new wave of robotic technology. They are called soft robots, and they look and function more like squishy insects and sea animals than they do R2-D2. That’s because their materials, systems, and processes are inspired by soft-structured creatures in nature. In the past decade or so, researchers have begun developing them to solve two major problems posed by traditional, rigid robots. One is the inability to adapt to and comply with surroundings. The other, related to the first, is the higher risk that comes into play when machines interact with humans.
A robotic arm on a factory assembly line, for example, is made for speed and precision. It excels at executing the same calculated task millions of times with minimal error. This capability is incredibly useful, especially for industrial applications, but this kind of robot’s high performance depends on a structured environment where its surroundings can be perfectly controlled. Conversely, a soft robot doesn’t need to be programmed for exact movements in known settings because it is designed to comply with and deform to its surroundings. Its elastic and rubbery parts can literally bend to a dynamic and complex environment, such as inside a human lung, where bronchi branch off in intricate patterns.
With the emergence and accessibility of digital fabrication technologies such as 3D printing, the field of soft robotics is advancing quickly. Researchers can build robot prototypes with greater structural complexity than ever before. This is Joyee’s expertise. “In additive manufacturing, this is the beauty of it,” she said. “You just change the design in SolidWorks. That’s it. It’s a one-time setup cost, and you can play with different material and use the same printing process.”
Over the past three years, Joyee has worked under the supervision of Assistant Professor Yayue Pan to develop a robot that can be printed in one piece and in one platform, without any assembly or pre- or post-processing. “There are so many robots in millimeter size which are able to deliver drugs, but the fabrication process of those robots is time-consuming—there are so many steps,” Joyee said. “We can print the whole robot in one step under one 3D-printing machine.”
What’s more, using novel manufacturing processes, Joyee and Pan have developed a robot with multiple unique features. They call the first feature “multi-material,” which enables them to give the robot prescribed material heterogeneity. This means that they can control the particle-polymer composite material locally, giving the robot enough magnetic strength in some regions and less in other regions to maintain its overall compliance. This helps the robot to mimic the natural inchworm locomotion and requires the least number of magnetic particles, a key factor in keeping it safe for human interaction.
In addition to being multi-material, their robot is multi-scale. Inspecting the robot under a microscope reveals hundreds of tiny hair-like bristles, and on each bristle, thousands of tinier holes. These structural components range from nanoscale to millimeter scale and give the robot its ability to deform according to the surface, whether it is smooth, uneven, dry, or damp. The combination of multi-material and multi-scale features allows the robot to carry a load up to 30 times its weight.
“There are so many robots in millimeter size which are able to deliver drugs, but the fabrication process of those robots is time-consuming—there are so many steps. We can print the whole robot in one step under one 3D-printing machine.”
PhD candidate, industrial engineering|
Most natural organisms possess multi-scale features to broaden their adaptive capabilities. For example, a gecko’s skin is covered in micro-spikes and nano-spores in each spike base to repel water and kill bacteria. Joyee and Pan have tapped into this paradigm to inform their material and device designs. Essentially, they can engineer virtually any known property achieved by surface structures in nature, from the light-harvesting power of a viola flower petal to a shark’s ability to reduce friction with its skin.
Unfortunately, 3D printing at this level of complexity isn’t something that you can do at home. “3D printers on the market today can only fabricate the same scale, or maybe two materials, but only in a uniform or graded way,” Pan said. But it’s most likely only a matter of time.
Robots like Joyee and Pan’s still face challenges. They must go through many clinical trials before becoming a viable option for medical applications, such as drug delivery. With further advancements in additive manufacturing and more collaboration to bring new robotic technologies to a broad array of applications, the future of robotics looks soft.